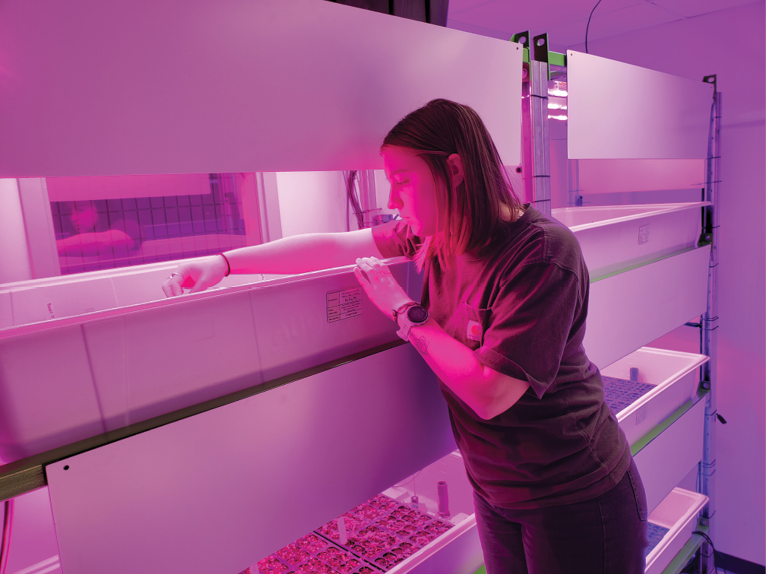
The Controlled Environment Agriculture Herb Extension & Research Base (CEA HERB) project team received a grant from the USDA to develop science-based information relevant to greenhouse and indoor production and economics of potted and fresh-cut culinary herbs produced in controlled environments. The team is led by Dr. Roberto Lopez at Michigan State University (MSU) and consists of faculty, researchers and graduate students at MSU, the University of Tennessee, North Carolina State University, Iowa State University, Texas Tech University and the USDA Agricultural Research Service.
The project objectives are to: 1. increase the demand and marketability of culinary herbs; 2. increase growth, quality, shelf life, disease management and food safety of herbs through environmental control and cultural practices; and 3. develop effective marketing, production, plant protection, technology adoption, post-harvest and food safety guidelines for CEA herb growers. More information and project updates are available online at scri-ceaherb.org.
Why herbs? First, consumer demand for fresh herbs has increased in the last decade. This has stimulated production in controlled environments to meet year-round demand, especially for holidays such as Thanksgiving. Second, little research-based information exists on these crops, at least partly because (until recently) they were a small production niche. Third, there are fewer pesticides labeled for herbs, which creates added challenge to manage diseases, insect pests and crop height. Finally, since culinary herbs are meant for consumption, food safety protocols are essential.
This article is the first of six to appear in Greenhouse Management in 2025. A complementary eight-part series will be published in sister publication Produce Grower (producegrower.com). In total, these 14 articles will provide research-based information on a variety of production, marketing and food safety topics focused on culinary herbs.
Here, we delve into light quality and how the light spectrum can be manipulated to regulate growth and especially crop height of herb transplants. Experiments were performed indoors to exploit potential light management opportunities. The objective was to determine how combinations of blue (400-499 nm), red (600-699 nm) and far-red (700-750 nm) light impact the growth and habit of young plants of culinary herbs. We focused on these light wavebands because previous research with lettuce and other leafy greens has shown that blue light inhibits leaf and stem expansion and thus growth, while far-red light does the opposite.

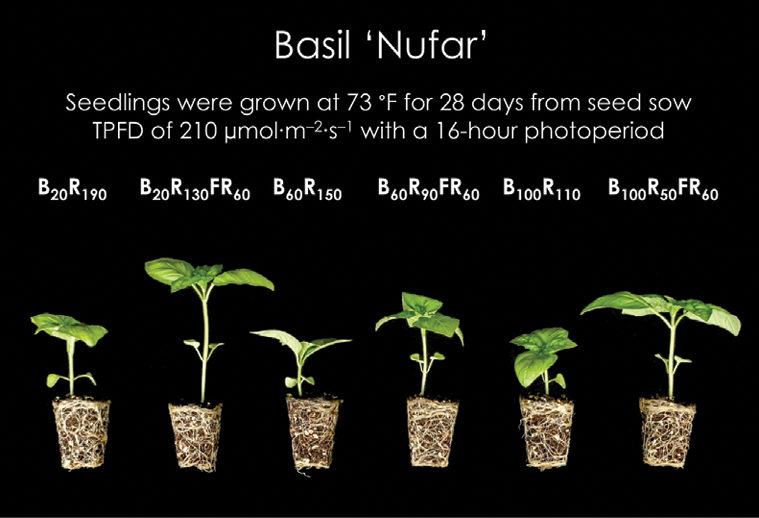
Experiment protocol
We sowed seeds of basil ‘Nufar’, cilantro ‘Santo’, parsley ‘Giant of Italy’, common sage, common mint and oregano ‘Greek’ in a peat-perlite substrate in 72-cell plug trays. Each cell had 2 to 4 seeds, depending on the species, and were thinned to 1 to 3 seedlings per cell: basil (1), cilantro (2), parsley (3), sage (2), mint (2) and oregano (3). Seeds germinated indoors at 73 °F (23 °C) under humidity domes under various lighting treatments that all had the same total photon flux density (light intensity) of 210 µmol∙m–2∙s–1. The seedlings were irrigated once daily with deionized water supplemented with a water-soluble 13N–3P–15 K fertilizer at an electrical conductivity of 0.43 dS∙m-1 and a pH of 5.5 to 6.0.
Six light spectrum treatments were created with Philips Green-Power LEDs and included 20, 60 or 100 µmol∙m–2∙s–1 of blue light, and either 0 or 60 µmol∙m–2∙s–1 of far-red light, with red light added to reach the target light intensity of 210 µmol∙m–2∙s–1 (Figure 1). All lighting treatments had a 16-hour photoperiod, and the temperature was maintained at 73 °F until harvest. These treatments are listed below, with B representing blue light, R representing red light and FR representing far-red light, and their subscripts denoting each color’s photon flux density in µmol∙m–2∙s–1:
B20 + R190
B20 + R130 + FR60
B60 + R150
B60 + R90 + FR60
B100 + R110
B100 + R50 + FR60
Plants were harvested when they could easily be removed from their plug trays without root breakage, which was approximately four weeks for basil, cilantro and parsley, and five weeks for sage, mint and oregano. At harvest, the following data were collected: shoot and root fresh weights, shoot and root dry weights, stem length, stem width (at its base), leaf number and leaf area. Stem length, stem width and leaf number were only collected for the largest plant per cell.
Specific leaf area was calculated by dividing the total leaf area by the shoot dry mass, which gives us an idea of how much mass was allocated to the leaves. For instance, a plant with a high specific leaf area generally has large and thin leaves, while a plant with a low specific leaf area has relatively small but thick leaves. The root dry mass fraction was calculated by dividing the root dry mass by the total dry mass of each plant. A large root dry mass fraction is often desirable for transplants because it is indicative of sturdiness, and plants with a large root dry mass fraction could be more resilient during the transplanting process.
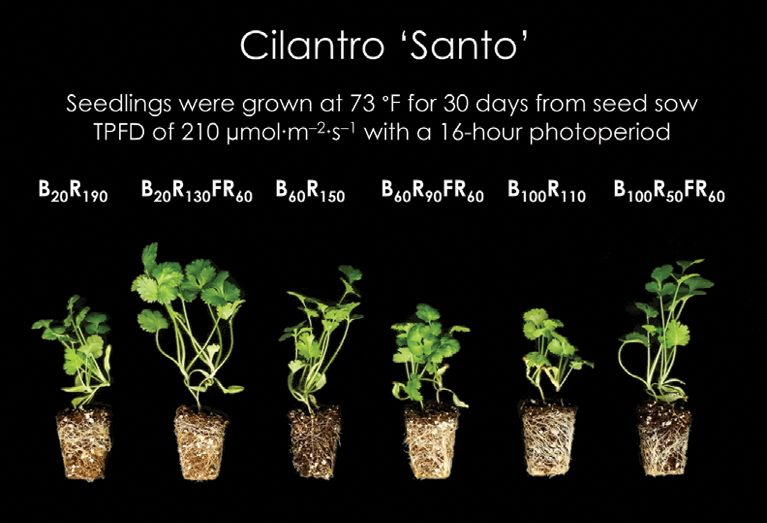
Results
Stem length
For all herb species, substituting red light with 60 µmol∙m–2∙s–1 of far-red light increased stem length. For example, basil stem length increased by 90 to 91% (Figure 2), and cilantro stem length increased by 66 to 75% (Figure 3). Increasing blue light had the opposite effect. For instance, without far-red light, increasing blue light from 20 to 60 µmol∙m–2∙s–1 decreased the stem length of all herb species. However, when combined with far-red light, increasing blue light from 20 to 60 µmol∙m–2∙s–1 decreased the stem length of only cilantro, parsley, sage and mint. Occasionally, increasing blue light from 60 to 100 µmol∙m–2∙s–1 continued to decrease stem length, but this only occurred in cilantro without far-red light, and sage with 60 µmol∙m–2∙s–1 of far-red light (Figure 4).
Root dry mass fraction
Far-red and blue light had opposite effects on the root dry mass fraction. For example, including far-red light in the spectrum decreased the root dry mass fraction (relatively less growth partitioned to the roots than shoots) of all herbs except oregano. In contrast, when far-red light was not in the spectrum, increasing blue light from 20 to 100 µmol∙m–2∙s–1 increased the root dry mass fraction (a relative increase in root growth) of parsley by 9 to 21% and sage by 16 to 26%.
Specific leaf area
Including far-red light in the spectrum increased the specific leaf area (i.e., larger and thinner leaves) of basil, cilantro, sage and parsley. In some cases, the increase in specific leaf area was the most pronounced under the highest blue light intensity. For instance, with 100 µmol∙m−2∙s−1 of blue light, substituting 60 µmol∙m−2∙s−1 of red light for far-red light increased the specific leaf area by 16 to 19% in cilantro and 13 to 21% in sage.
Shoot dry mass
Including far-red light in the spectrum increased shoot growth of basil, cilantro and mint, as indicated by the shoot dry mass. In some cases, the increase in shoot dry mass was the most pronounced under the highest blue light intensity. For instance, with 100 µmol∙m−2∙s−1 of blue light, substituting 60 µmol∙m−2∙s−1 of red light for far-red light increased the shoot dry mass of cilantro by 36 to 47%.
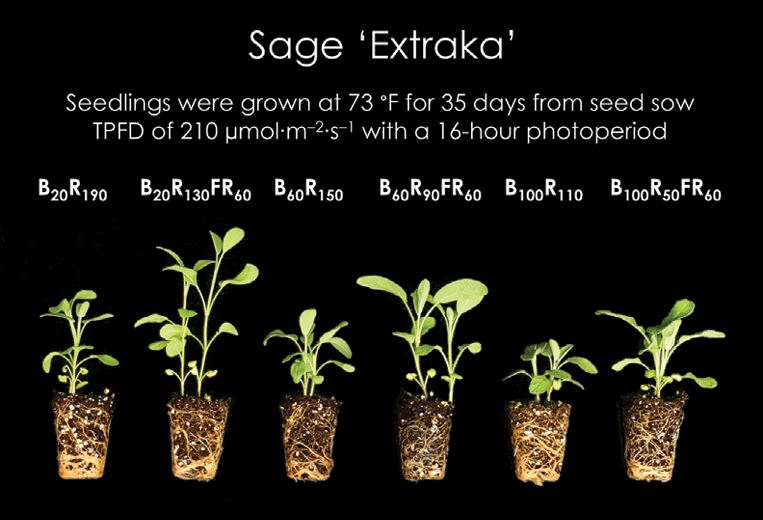
Take-home messages
While the interactions between blue and far-red light were often variable, the effects of blue and far-red light on their own were much clearer.
Far-red light generally increased stem length, shoot dry mass and specific leaf area, but decreased the root dry mass fraction. Blue light decreased stem length and occasionally increased the root dry mass fraction.
In the context of producing high-quality transplants of culinary herbs, including far-red light in the spectrum decreased transplant quality by reducing sturdiness and compactness, while increasing blue light (up to 60 µmol∙m−2∙s−1) increased transplant quality by doing the opposite. However, increased shoot dry mass and specific leaf area (with far-red light) may be of higher priority for certain herb species, especially after transplant.
In many cases, the effects of far-red light were the most dramatic under the highest blue light intensity: 100 µmol∙m−2∙s−1. However, the effects of far-red light are predominantly mediated by the red to far-red light ratio, not just the far-red light intensity.
With this in mind, an increase in the blue light intensity was offset by a decrease in red light to maintain the same total light intensity. This increased the red to far-red light ratio, which could explain the more dramatic increases in specific leaf area and shoot dry mass under high blue light.
Therefore, more research is needed to separate the specific effects of blue light from far-red light.
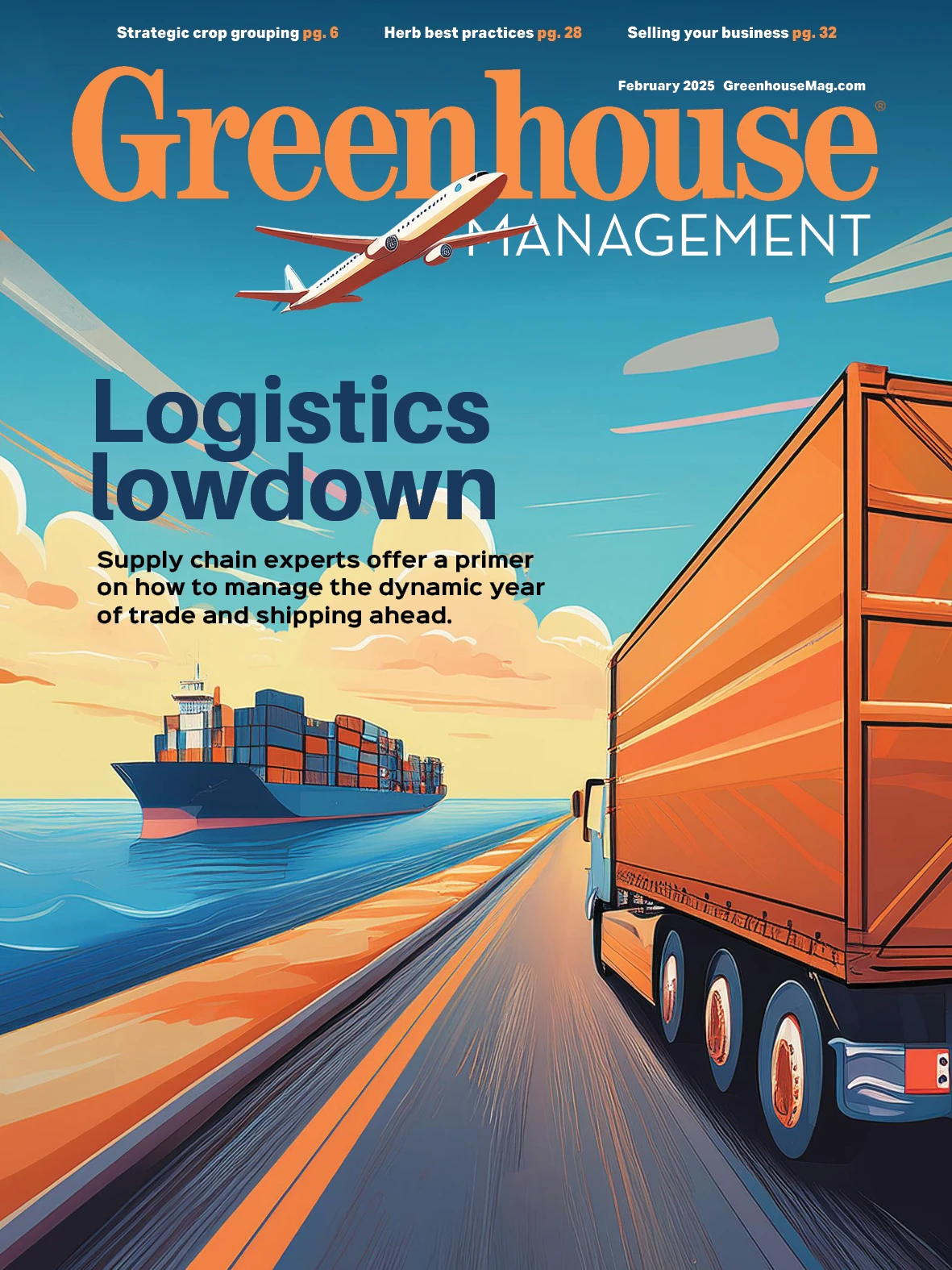
Explore the February 2025 Issue
Check out more from this issue and find your next story to read.
Latest from Produce Grower
- Mind matters
- John Bonner focuses on purposeful progress as founder of Great Lakes Growers
- The Growth Industry Episode 1: State of the Horticulture Industry
- FDA to Hold Webinar on Updated ‘Healthy’ Claim
- VIDEO: Growing media for strawberries grown under different production systems
- Eden Green Technology CEO Eddy Badrina reflects on challenges, opportunities for CEA
- Why CEA businesses should track carbon KPIs
- UGA professor Erich Schoeller to discuss IPM best practices for CEA at Indoor Ag-Con 2025